Radiometric dating
Radiometric dating, radioactive dating or radioisotope dating is a technique used to date materials such as rocks, minerals and organic remains (carbon), into which radioactive impurities were selectively incorporated when they formed. The method is based on comparing the abundance of a naturally occurring radionuclide within the material with the abundance of its decay products, which form at a known constant rate of decay. The use of radiometric dating was first published. time in 1907 by the American radiochemist Bertram Boltwood (1870-1927) —from an idea already advanced by the British Ernest Rutherford in 1905— and is now the main source of information on the absolute age of rocks and other geological characteristics, including the age of fossilized life forms or the age of the Earth itself, and can also be used to date a wide range of natural materials and ancient artifacts. By allowing the establishment of geologic time scales, it provides an important source of information about the ages of fossils and deduced rates of evolutionary change.
Along with stratigraphic principles, radiometric dating methods are used in geochronology to establish the geologic time scale. Among the best-known techniques are potassium-argon dating, uranium-lead dating, and radiocarbon dating (based on in the decay of the carbon-14 isotope), commonly used for dating relatively recent organic remains, up to 60,000 years. (Other possible datings are K/ Ar, U/Pb, Rb/Sr, Sm/Nd, etc.)
The different methods of radiometric dating vary according to the time scale in which they are precise and the materials to which they can be applied.
Basics
Radioactive decay
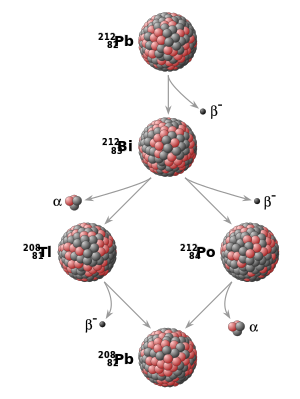
All ordinary matter is made up of combinations of chemical elements, each with its own atomic number, which indicates the number of protons in the atomic nucleus. Furthermore, elements can exist in different isotopes, with each isotope of an element differing in the number of neutrons in the nucleus. A particular isotope of a particular element is called a nuclide. Some nuclides are inherently unstable. That is, at some point in time, an atom of such a nuclide will undergo radioactive decay and spontaneously transform into a different nuclide. This transformation can be accomplished in a number of different ways, including alpha decay (emission of alpha particles) and beta decay (emission of electrons, positron emission, or electron capture). Another possibility is spontaneous fission into two or more nuclides.
Although the moment at which a particular nucleus decays is unpredictable, a collection of atoms of a radioactive nuclide decays exponentially at a rate described by a parameter known as a half-life, usually given in units of years when studying the dating techniques. After one half-life has elapsed, half of the atoms of the nuclide in question will have decayed into a "daughter" nuclide or decay product. In many cases, the "daughter" nuclide itself is radioactive, resulting in a new decay chain, ultimately ending with the formation of a stable (non-radioactive) "daughter" nuclide; each step in such a chain is characterized by a different half-life. In these cases, the half-life of interest in radiometric dating is generally the longest in the chain, which is the rate-limiting factor in the eventual transformation of the radioactive nuclide into its stable 'child'. Isotopic systems that have been exploited for radiometric dating have half-lives ranging from only about 10 years (for example, tritium) to more than 100 billion years (for example, samarium 147).
For most radioactive nuclides, the half-life depends solely on nuclear properties and is essentially a constant. It is not affected by external factors such as temperature, pressure, the chemical environment, or the presence of an electric or magnetic field. The only exceptions are nuclides that decay by the process of electron capture, such as beryllium 7, strontium 85 and zirconium 89, whose decay rate can be affected by the local electron density. For all other nuclides, the ratio of the parent nuclide to its decay products changes in a predictable manner as the parent nuclide decays over time. This predictability allows the relative abundances of related nuclides to be used as a clock to measure the time from the incorporation of the original nuclides into a material to the present.
Precision of radiometric dating
The basic equation of radiometric dating requires that neither the "parent" nuclide nor the "daughter" product can enter or leave the material after its formation. The possible confounding effects of contamination of the 'parent' and 'daughter' isotopes must be considered, as should the effects of any loss or gain of these isotopes since the sample was created. Therefore, it is essential to have as much information as possible about the material being dated and to check for signs of alteration. Accuracy is improved if measurements are taken on multiple samples from different locations in the rock body. Alternatively, if several different minerals can be dated from the same sample and are assumed to have formed by the same event and were in equilibrium with the reservoir when they formed, they should form an isochron. This can reduce the contamination problem. In uranium-lead dating, the concord diagram is used which also alleviates the problem of missing nuclides. Finally, the correlation between the different isotopic dating methods may be necessary to confirm the age of a sample. For example, the age of the Amitsoq gneisses in western Greenland was determined to be 3.6 ± 0.05 million years (Ma) using lead-uranium dating and 3.56 ± 0.10 Ma using lead-uranium dating. lead-lead, results agreeing with each other.
Accurate radiometric dating generally requires that the "parent" have a half-life long enough for it to be present in significant amounts at the time of measurement (except, as described below, in "Radionuclide Dating"). short-lived extincts"), that the half-life of the "parent" is precisely known, and that sufficient quantity of the "daughter" product is produced to measure and distinguish it from the initial quantity of the "daughter" present in the material. The procedures used to isolate and analyze the "parent" and "daughter" nuclides must be accurate and reliable. This typically involves the use of isotope ratio mass spectrometry.
The accuracy of a dating method depends in part on the half-life of the radioactive isotope involved. For example, carbon 14 has a half-life of 5730 years. After an organism has been dead for 60,000 years, so little carbon-14 remains that precise dating cannot be established. On the other hand, the carbon-14 concentration drops so steeply that the age of relatively young remains can be determined to within a few decades.
Closing temperature
If a material that selectively rejects the daughter nuclide is heated, any daughter nuclides that have accumulated over time will be lost by diffusion, resetting the isotopic clock to zero. The temperature at which this happens is known as the closing temperature or blocking temperature and is specific to each particular material and isotopic system. These temperatures are determined experimentally in the laboratory by artificially resetting sample minerals using a high-temperature furnace. As the mineral cools, the crystalline structure begins to form and diffusion of isotopes becomes less easy. At a certain temperature, the crystal structure has formed enough to prevent isotope diffusion. That temperature is what is known as the closure temperature and represents the temperature below which the mineral is a closed system for isotopes. Therefore, an igneous or metamorphic rock or melt, which is cooling slowly, does not begin to show measurable radioactive decay until it cools below the shut-in temperature. The age that can be calculated by radiometric dating is therefore the time when the rock or mineral cooled to the closing temperature. Dating different minerals and/or isotope systems (with different cooling temperatures) closure) within the same rock can therefore allow the tracking of the thermal history of the rock in question over time, and therefore the history of metamorphic events can be known in detail. This field is known as thermochronology or thermochronometry.
Dating equation
The mathematical expression that relates radioactive decay to geological time is:
D=D0+N(t)(eλ λ t− − 1){displaystyle D=D_{0}+N(t)(e^{lambda t}-1)}
or
t=1λ λ ln (1+DD0){displaystyle t={frac {1}{lambda }{ln left(1+{frac {D}{D_{0}}}}}{right)}}}}}
being:
- t={displaystyle t=}, the age of the sample,
- D={displaystyle D=}the number of atoms of the isotope “son” in the sample,
- D0={displaystyle D_{0}=}, the number of atoms of the isotope "son" in the original composition (equal to the number of "father" atoms that have decayed radioactively),
- N(t){displaystyle N(t)}the number of atoms of the isotope "father" in the sample at the time t (the present), given by N(t) = Nore-λtand
- λ λ ={displaystyle lambda =}the constant radioactive disintegration of the “father” isotope, equal to the reverse of the average life—average duration of a radioactive isotope prior to its disintegration—of the “father” isotope multiplied by the neperian or natural logaritmo (or natural) (ln{displaystyle ln }) of 2. The average life is equal to the reverse of the radioactive disintegration constant: Δ Δ =1/λ λ {displaystyle tau =1/lambda ,!}. As long as it happens until the amount of nucleides is reduced to half of the initial amount is called a period of semi-disintegration, period, semi-period or semi-life (not to confuse with the average life): T1/2=ln(2)/λ λ {displaystyle T_{1/2}=ln(2)/lambda ,!}. At the end of each semi-disintegration period, the radiation is reduced to half (from the initial). Each radioisotope has a characteristic semi-disintegration period, in general different from the respective of other isotopes.
Main data | |||
Isótopo | Period | Emission | |
---|---|---|---|
Radom 222 | 3.8 days | Alpha | |
Cobalt 60 | 5271 years | Gamma | |
Carbon 14 | 5730 years | Beta | |
Uranium 238 | 4510 million years | Alpha |
The equation is best expressed in terms of the measured amount N(t){displaystyle N(t)} instead of the constant initial value N0{displaystyle N_{0}}.
The above equation makes use of information about the composition of the "parent" and "daughter" isotopes at the time the material under test cooled below its shut-off temperature. This is well established for most isotopic systems. However, construction of an isochrone does not require information about the original compositions, simply using the current ratios of the "parent" and "child" isotopes of a standard isotope. Plotting an isochron is used to graphically solve the age equation and calculate the age of the sample and the original composition.
This equation is valid as long as the decay mode of the “parent” isotope is unique and the “daughter” isotope is stable. For other cases, more complex equations can be obtained, in which multiple possible decays are taken into account.
Modern dating methods
Radiometric dating has been known since 1905, when it was devised by Ernest Rutherford as a method by which the age of the Earth could be determined. In the century since, techniques have been greatly improved and expanded. Dating can now be performed on very small samples, on the order of a nanogram, using a mass spectrometer. The mass spectrometer was invented in the 1940s and began to be used for radiometric dating in the 1950s. It operates by generating a beam of ionized atoms from the sample under test. The ions then travel through a magnetic field, which deflects them toward different sampling sensors, known as "Faraday cups," depending on their mass and level of ionization. Upon impact with the cups, the ions establish a very weak current that can be measured to determine the rate of impact and the relative concentrations of the different atoms in the beams.
Uranium-lead dating method
Uranium-plom dating (U-Pb) is one of the oldest and most refined radiometric dating systems. It can be used to date rocks that have formed and crystallized for 1 million years up to 4500 million years with precisions within the percentage range of 0.1-1%, less than two million years in 2500 million years. There has been a margin of error of 2–5% in the younger mesozoic rocks.
The uranium-plom dating method is based on two disintegration chains, the uranium series 238U a 206Pb, with an average life time of 4470 million years and the show 235U a 207Pb, with an average lifetime of 704 million years. That is one of its great advantages, which any sample provides two watches, which provides an incorporated cross check that allows to accurately determine the age of the sample, even if part of the lead has been lost. This can be seen in the concord diagram, where the samples are traced along an error chron (errorchron) (right line) that crosses the concord curve in the age of the sample.
U-Pb dating is often done in the mineral zircon (ZrSiO4), although it can be used in other materials, such as baddeleyite, as well as monazita (see: monazita geochronology). The zirconium and the baddeleyite incorporate uranium atoms into its crystalline structure as substitutes for zirconium, but reject the lead. The circonium has a very high closing temperature, is resistant to mechanical weather and is very chemically inert. The zirconium also forms multiple layers of crystal during metamorphic events, each of which can record an isotope age of the event. Microhaz analysis in situ can be achieved through ICP-MS or SIMS laser techniques.Samarium-Neodymium dating method
The samario-neodimio dating or dated via samario-neodimio is a useful radiometric dating technique to determine the ages of rocks and meteorites. The technique is based on the decay of the samario isotope (Sm), which implies an alpha decay from 147Sm to 143Nd with an average life of 106 billion years. The content of the Nd isotope is used to provide information about the source of igneous material as well as its antiquity. The various deposits in the solid parts of the Earth have different values from the initial relationships of 143Nd/144Nd, especially regarding the mantle.
The usefulness of the Sm-Nd dated technique is based on the fact that these two elements are rare lands. Therefore, theoretically they are not susceptible to separate during the fusion of silicate rocks. Fractioned crystallization effects of ferlsic minerals change the Sm/Nd ratio of the resulting materials. This in turn influences the relationship 143Nd/144Nd with the increase 143Nd.
The Earth mantle was supposed to have a condritic evolution and, therefore, that it departed from the initial relations of 143Nd/144Nd what can provide information about the age at which a particular rock or a deposit separated from the mantle in the past of the Earth.
Precision levels of twenty million years can be reached in the ages of two thousand five hundred million years. In many cases, information obtained through Sm-Nd and Rb-Sr techniques is used in a complementary manner.Potassium-argon dating method
The potassium-argon dating 40K/40Ar is a method of radiometric dating that emerged in the 1960s and is used in geology and archaeology to date volcanic rocks or ashes, in general, the oldest.
It is based on the principle of radioactive decay, and involves the capture of electrons or the decomposition of positrons of the potassium radioactive isotope 40 (40K) —presents in the volcanic rocks — which disintegrates at a rhythm known in the inert gas argon 40 (40Ar), in a process that has an average life of (1248 million years) time during which the gas is concentrated on the crystals of the rock. Taking advantage of that known average rhythm and life, the method is lent to data samples ranging from the 100 000 years up to several billion years. The limit for younger samples has been set to control the measurement error by argon incorporations from the atmosphere. Radioactive 40K is common in micas, feldspartos and bakery, although the closing temperature is fairly low in those materials, approximately 350 °C (mica) to 500 °C (hornblenda).
A remarkable refinement of this technique was obtained in 1965 by radiation of samples into a nuclear reactor to transform stable potassium 39K in the isotope 39Ar. This method was called 40Ar/39Ar, a procedure that — albeit much more expensive — is ten times more accurate, with an error rate of about 1%.Rubidium-strontium dating method
The dating method by rubdium-srontium is a radiometric dating technique used to determine the age of rocks and minerals from the quantities they contain from the rubid isotopes (87Rb) and strontium (87Mr.86Mr.
This method is based on the beta disintegration of the 87Rb (one of the two isotopes of the rubidio that are presented naturally) that decays to the 87Sr with a semi-deintegration period of 48 800 million years. In addition, rubidium is a highly incompatible element that, during the fragmented crystallization of the earth's crust, remains in the melted magmatic mixture instead of being part of the minerals of the mantle. The radiogenic “son” 87Mr, produced in this process of decay, was also produced in the stellar nucleosynthesis cycles prior to the creation of the solar system.
Different minerals in a certain geological environment may have over time different ratios of 87 radiogenic strontium with respect to the 86 stront in nature, so that its age can be determined by measuring 87Mr.86Mr with a mass spectrometer, from knowing the amount of 87Mr that existed when the rock or mineral was formed, and calculating the amount of 87Rb from measuring the rubid content and mass ratio 85Rb/87Rb.
If these minerals crystallized from the same molten mass of silicates, each mineral had the same initial relation 87Mr.86Sr que su «padre» en la masa fundida. However, since rubid is replaced by potassium in minerals and these minerals have different K/Ca relationships, minerals will have different Rb/Sr relationships.
During the fractional crystallization, Mr tends to concentrate on plagioclase, leaving the ruby in the liquid phase. Therefore the Rb/Sr ratio in the residual magma can increase over time, giving rise to rocks with higher Rb/Sr relationships with increasing differentiation. High relationships (from 10 or more) occur in the pegmatites. Generally, the Rb/Sr ratio increases in plagioclase, bakery, potassium feldspartos, biotite, moscovita. Therefore, given sufficient time for significant production (internal growth) of 87Radiogenic measured values 87Mr. 86Mr will be different in minerals, increasing in the same order.
This scheme is used to date ancient igneous and metamorphic rocks, and has also been used to date lunar samples. Closing temperatures are so high that they are no cause for concern. Ruby-state dating is not as accurate as uranium-plom dating, with errors of 30-50 million years for a sample of 3000 million years.
The development of this technique was the product of the work of the German chemical Otto Hahn and Fritz Strassmann, who would then discover the nuclear fission in December 1938.Uranium-thorium dating method
The uranium-torium dating, also called torium 230 dating, dating from uranium series imbalance or uranium series dating, is a radiometric dating technique commonly used to discover the age of materials formed by calcium carbonate, such as speleothemes or corals. Unlike other commonly used radiometric dating techniques that date the build-up of a stable final member from disintegration—such as those of rubid-state or uranium-plom—, the uranium-tory technique does not and instead calculates an age from the degree to which secular balance has been restored between the radioactive isotope 230Th and his father 234U inside a sample.
The torium is not soluble in the natural waters in the conditions that are in, or near, the surface of the earth, so the materials formed in, or from, these waters do not usually contain torium since it is precipitated selectively in the sediments of the bottom of the ocean, from which its proportions are measured. In contrast, uranium is soluble to some extent in all natural waters, so any material that precipitates or forms from such waters also contains traces of uranium, typically at levels of a few parts per billion and a few parts per million, in weight. As time passes after material formation, the 234U present in the sample, with an average life of 245 000 years, decays 230Th. The torium-230 is itself radioactive, with an average life 75 000 yearsso they do not accumulate indefinitely (such as the case of uranium-plome of the system), 230Th instead approaches secular balance with his radioactive father 234U. In secular balance, the amount of 230Th that disintegrates per year within a sample is equal to the amount of torium-230 produced, which is also equal to the amount of 234U that disintegrates per year in the same sample.
dating based on uranium-torio has a higher age limit of some more 500 000 yearswhich is established by the average life of 230Th, for the accuracy with which the relationship can be measured 230Th/234U in a sample and for the accuracy with which the average life of the 230Th and the 234U. It should also be borne in mind that to calculate an age with this technique the proportion of 234U and his isotope "father", the 238U, it must be measured.
A relatively short range dating technique is based on decomposition of the 234U 230Th, a substance with an average life of approximately 80 000 years. It is accompanied by a brotherly process, in which the 235Th disintegrates in protactinio 231, which has an average life 32 760 years which is also water-solubleRadiocarbon dating method
Radiocarbon dating is a radiometric dating method that uses the carbon-14 radioactive isotope (carbon-14).14C) to determine the age of carbon-containing materials to some 50 000 years. The 14C has an average life 5730 years(which is very short compared to other isotopes used in radiometric dating) and decomposes in nitrogen. In other dating methods, the heavy parental isotopes were produced by nucleosynthesis in supernovas, which means that any parental isotope with a short average life should already be extinct. The 14C, however, is continually created through the collisions of neutrons generated by cosmic rays with nitrogen in the upper atmosphere and therefore remains at an almost constant level on Earth. The 14C ends as a trace component in atmospheric carbon dioxide (CO)2).
A carbon-based lifestyle incorporates carbon continuously throughout its lifetime. The plants acquire it through photosynthesis, and the animals, through the consumption of plants and other animals. When an organism dies, it stops absorbing again 14C and the existing isotope decay according to their characteristic half-life. The proportion of 14C that remains when the body remains are examined provides an indication of the time elapsed since his death. This makes the 14C is an ideal method to date the age of the bones or the remains of an organism. The data limit by 14C is between 58 000 years and 62 000 years.
Creation rate 14C appears to be approximately constant, as cross-checks of dating by 14C with other dating methods show that it gives consistent results. However, local eruptions of volcanoes or other events that emit large amounts of carbon dioxide can reduce local concentrations 14C and give inaccurate dates. Carbon dioxide emissions to the biosphere as a result of industrialization have also reduced the proportion 14C in a small percentage; and the reverse, the amount 14C increased by the surface testing of nuclear bombs conducted in the early 1960s. In addition, an increase in the solar wind or magnetic field of the Earth above the current value would depress the amount 14C created in the atmosphere.
In archaeology it is considered an absolute dating technique. In 1946, American chemist Willard Libby announced the formation mechanisms of the isotope 14C through nuclear reactions in the atmosphere. Later, in 1949, when he served as a professor at the University of Chicago, he developed the method known as a method of radiocarbon dating. In 1960, Libby was awarded the Nobel Prize in Chemistry for its dating method 14C.Fission track dating method
This method involves inspecting a polished cut of a material to determine the density of the "trace marks" (track) left in it by the spontaneous fission of uranium-238 impurities. The uranium content of the sample must be known, but that can be determined by placing a plastic film over the polished slice of the material and bombarding with slow neutrons. This causes induced fission of 235U, as opposed to spontaneous fission of 238U. The fission tracks produced by this process are recorded on the plastic film. The uranium content of the material can be calculated from the number of traces and the neutron flux.
This scheme has application over a wide range of geologic dates. For dates up to a few million years, micas, tektites (glass fragments from volcanic eruptions), and meteorites are best used. The oldest materials can be dated using zirconium, apatite, titanite, epidote, and garnet which have varying amounts of uranium content. Because fission tracks are cured at temperatures in excess of 200 °C, the technique has both limitations as benefits. The technique has potential applications for detailing the thermal history of a reservoir.
Chlorine 36 dating method
Between 1952 and 1958, large amounts of the otherwise rare 36Cl (half-life ~ 300 ka) were produced during the atmospheric detonation of nuclear weapons. The residence time of 36Cl in the atmosphere is approximately 1 week. Therefore, as a marker of 1950s soil water and groundwater events, 36Cl is also useful for dating water less than 50 years before present. 36Cl has been used in other areas of geological sciences, such as dating ice and sediments.
Luminescence dating methods
Luminescence dating methods are not radiometric dating methods because they do not rely on isotope abundance to calculate age. Instead, they are a consequence of background radiation in certain minerals. Over time, ionizing radiation is absorbed by mineral grains in sediments and archaeological materials such as quartz and potassium feldspar. The radiation causes charge to remain within the grains in structurally unstable "electron traps." Exposure to sunlight or heat releases these charges, which "bleachs" the sample and resets the clock to zero. The trapped charge accumulates over time at a rate determined by the amount of background radiation at the location where the sample was buried. Stimulation of these mineral grains by light (optical stimulated luminescence or infrared stimulated luminescence dating) or heat (thermoluminescence dating) causes a luminescence signal to be emitted as stored unstable electronic energy is released, the intensity of which it varies depending on the amount of radiation absorbed during burial and the specific properties of the mineral.
These methods can be used to date the age of a layer of sediment, since layers deposited on top would prevent the grains from "bleaching" and were restored by sunlight. Pottery shards can be dated to the last time they experienced significant heat, usually when they were kiln-fired.
Thermoluminescence dating methods
Termoluminiscent dating is an absolute dating method used in archaeology to determine the age of elements that have been subjected to heating, such as homes or ceramics. It can also be used to date wind, fluvial, marine, coastal, volcanic rocks and precipitated calcium carbonate in caves. It is based on the alterations caused by ionizing radiation (cosmic radiation and radiation from the environment) in the crystalline structures of minerals, increasing thermalescence of these with the time of exposure to radiation. There are several ways to make this type of dating, highlighting: "pre-dosis" technique, "sleep grain" and "inclusions".
To be able to use this test it is necessary that the element that will be dated (ceramic, furnace...) contains thermal minerals (usually quartz) and that has been subjected to a temperature greater than 500 °C. In the case of sediments, these have to be exposed for some time to sunlight. Samples analyzed must be between 1,000 and 500 000 years old.Other methods
Other methods include:
- argon-argon data (Ar-Ar)
- Iodo-xenon data (I-Xe)
- Plain-barium data (La-Ba)
- lead-plome data (Pb-Pb)
- dating lutecio-hafnio (Lu-Hf)
- dating potassium-calcium (K-Ca)
- dating renio-osmio (Re-Os) (Re-Os)
- uranium-plom dating - helium (U-Pb-He)
- uranium-uranium (U-U) data
- cryption-cryption data] (Kr-Kr)
- berilio (10Be-9Be)
Dating with decay products of short-lived extinct radionuclides
Absolute radiometric dating requires that a measurable fraction of the "parent" core remain in the sample rock. For rocks dating back to the beginning of the solar system, this requires extremely long-lived "parent" isotopes, making measurement of the exact ages of such rocks imprecise. In order to distinguish the relative ages of the rocks from that ancient material and to obtain better temporal resolution than is available from long-lived isotopes, short-lived isotopes no longer present in the rock, known as extinct radionuclides, can be used.
At the beginning of the solar system, there were several relatively short-lived radionuclides such as 26Al, 60Fe, 53Mn, and 129I present in the solar nebula. These radionuclides, possibly produced by a supernova explosion, are now extinct, but their decay products can be detected in very old material, such as meteorites. By measuring the decay products of extinct radionuclides with a mass spectrometer and using isocronplots, it is possible to determine the relative ages of different events in the early history of the solar system. Dating methods based on extinct radionuclides can also be calibrated with the U-Pb method to obtain absolute ages. Therefore, both approximate age and high time resolution can be obtained. In general, a shorter half-life leads to higher time resolution at the expense of time scale.
The Chronometer 129I – 129Xe
The 129
I decays to 129
Xe with a half-life of 16 million years. The iodine-xenon chronometer is an isochronous technique. The samples are exposed to neutron radiation in a nuclear reactor. This makes the only stable isotope of iodine (129
I) in 128
Xe via neutron capture followed by beta decay (from the 128
I). After irradiation, the samples are heated in a series of steps and the isotopic signature of xenon in the evolved gas is analyzed in each step. When observing a constant ratio of 129
Xe / 128
Xe in several consecutive temperature steps, can be interpreted as corresponding to a moment in which the sample stopped losing xenon.
Samples from a meteorite called Shallowater are typically included in the irradiation to monitor the conversion efficiency of 127
I a 128
Xe. The difference between the measured ratios of 129
Xe / 128
Xe of the sample and of the Shallowater correspond to the different ratios of 129
I/127
I when each of them stopped losing the xenon. This, in turn, corresponds to a difference in the closing age in the early solar system.
The chronometer 26Al – 26Mg
Another example of dating short-lived extinct radionuclides is the 26Al – 26Mg chronometer, which can be used to estimate the relative ages of chondrules. 26Al decays to 26Mg with a half-life of 720,000 years. Dating is simply a matter of finding the deviation of the natural abundance of 26Mg (the decay product of 26Al) compared to the stable isotope ratio 27Al/24Mg.
The excess of 26Mg (often designated 26Mg*) is found by comparing the ratio of 26Mg/27 Mg with that of other materials in the solar system.
The 26Al – 26Mg chronometer gives an estimate of the time period for the formation of primitive meteorites of only a few million years (1.4 million of years for the formation of the chondrule).
Contenido relacionado
Ténéré desert
Arnold sommerfeld
Redshift